In recent years, the field of metamaterials has experienced substantial growth, revealing exciting potential, especially in advancing magnetic resonance imaging (MRI) technology. Three new studies led by Dr. Xin Zhang, a BU College of Engineering Distinguished Professor and a professor at the BU Photonics Center, highlight the promising opportunities within this field.
These studies, in collaboration with Dr. Stephan Anderson, a BU Chobanian & Avedisian School of Medicine professor of radiology, published in Advanced Science, Advanced Materials, and Science Advances, showcase innovative approaches to enhance the MRI experience for all patients.
From integrating metamaterials with computer-aided embroidery technology to the development of wireless, lightweight coils adaptable to 3D curved body contours using coaxial cables, each paper offers its own distinct strategy. In this Q&A, Dr. Zhang discusses how her work is shaping the future of MRI technology by blending comfort, precision, and affordability.
What are metamaterials, and how do the research findings discussed in the Advanced Science, Advanced Materials, and Science Advances articles suggest they can enhance MRI technology?
Metamaterials, constructed from assemblies of multiple precisely designed structures at the subwavelength scale, have emerged as a powerful tool for tailoring the effective properties of materials by manipulating the amplitude, phase, and polarization of propagating waves. These materials have found widespread applications, including cloaking devices, perfect absorbers, wireless power transfer, and high-sensitivity sensing.
Due to their unique capacity for electromagnetic field confinement and enhancement, metamaterials offer a new perspective on boosting the imaging performance of MRI in a wireless and cost-effective manner.
Briefly, metamaterials are composed of arrays of unit cells featuring electromagnetic resonators, whose coupling leads to synergy and a collectively resonating mode. In the context of MRI, this synergy ultimately leads to a dramatic increase in the signal-to-noise ratio (SNR) of MRI and thus significantly improves the performance of MRI.
What is new and noteworthy about your findings?
In this work, we pioneered the use of off-the-shelf coaxial cables to construct form-fitting coils and conformal metamaterials for MRI applications. Coaxial cables, characterized by an inner conductor surrounded by a concentric conducting shield, with the two separated by a dielectric layer, provide efficient signal transmission and protection against external interference.
By minimizing dielectric loss and capacitive detuning, these delicately designed resonators can preserve a high quality factor and demonstrate robustness against loading variations when imaging conducting samples in an MRI system. In contrast to previously reported MRI metamaterials, the coaxially-shielded metamaterials offer a substantial SNR gain due to their remarkable magnetic response and the suppressed electric dipole moment found in conventional metamaterials.
Leveraging the inherent flexibility and electric field-confining properties of coaxial cables, these coils function wirelessly as additive components with the body coil, enhancing MRI imaging power.
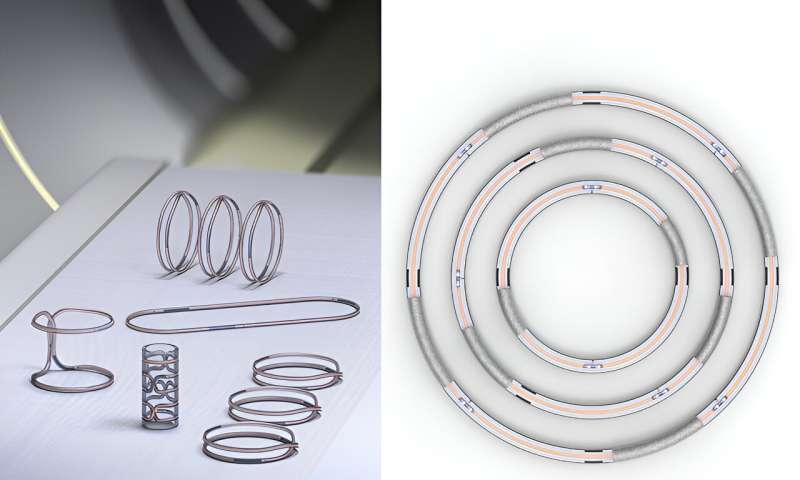
Your Advanced Materials study introduces the concept of wireless MRI coils made from coaxial cables. How do these coils address the limitations of traditional coil arrays, and what advantages do they offer?
For many years, conventional anatomy-specific RF receive coils have been routinely used in MRI to provide high sensitivity in signal acquisition. However, their bulky, fixed, and rigid configurations often lead to patient discomfort, difficult positioning, and compromised signal sensitivity in certain scenarios.
Additionally, the necessity for specific coil designs targeting particular anatomical areas has resulted in imaging centers maintaining 5–7 separate coils, significantly increasing costs.
To address these challenges, our research introduces a groundbreaking solution — wireless, lightweight, coaxially-shielded and conformal metamaterials that can accommodate anatomies of various sizes.
The proposed coaxial resonators demonstrate versatility by functioning both independently in form-fitting configurations, closely adapting to relatively small anatomical sites, and collectively by inductively coupling together as metamaterials. This allows for the extension of the field-of-view (FOV) coverage to encompass larger anatomical regions.
In what ways do the coil designs presented in the Science Advances study enhance patient comfort during MRI scans?
The coils presented in Science Advances enhance patient comfort in three key aspects. Firstly, the wireless design of these coils reduces positioning constraints and requires fewer adjustments, allowing for greater flexibility in patient positioning while maintaining signal strength and image quality.
Secondly, the coils are designed in compact and form-fitting configurations, enabling them to be easily snapped or placed onto the arm, wrist, or ankle without the need for additional tools or fasteners. Lastly, the ultra-lightweight nature of these coils, with the proposed coaxial coils weighing only tens of grams, further enhances patient comfort.
Are there any challenges associated with implementing the technologies described in these papers? If so, how might you address them in future studies?
The current fabrication of coils and metamaterials relies on 3D printing, embroidery machines, and manual soldering and assembly. While these methods are convenient for iterative design refinement, they may be inefficient for large-volume production and potential commercialization. An improvement could involve upgrading the fabrication process to utilize automatic coil winding systems and plastic injection molding.
Future endeavors should focus on improving the tuning process for tunable coils. A potential improvement involves connecting these coils wirelessly to the MRI system to form an efficient feedback loop, achieving automatic and precise frequency matching. A limitation of note of the proposed coils is that they may not be compatible with parallel imaging due to their cable-free design.
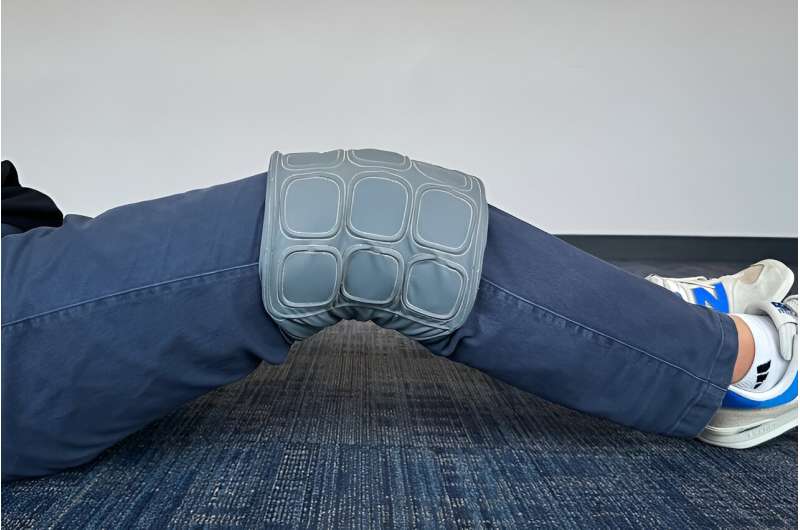
However, the proposed coils could provide a comparable or even better SNR than current commercially available surface coils at extremely low cost. The inexpensive, highly customizable coils have great potential of increasing the availability of lower cost MRI to society and finding many diverse applications throughout the MRI landscape.
How do the findings of these research papers pave the way for the next generation of MRI technology, and what impact could this have on clinical practice and patient care?
The ideal next-generation MRI coil would combine improved image quality, enhanced patient comfort, increased adaptability, and ease of implementation in clinical settings.
Capitalizing on the coaxial cable’s minimized dielectric loss and optimal form-fitting design, these wireless conformal coils and metamaterials exhibit heightened sensitivity in signal acquisition.
In addition to providing high image quality, these wireless, lightweight, and conformal coils enhance patient comfort and streamline the implementation process, ultimately improving patient throughput and overall efficiency in the imaging department.
Furthermore, facilitated by metamaterials technology, these coils offer a solution to wirelessly assemble multiple coils for customizable field-of-view (FOV) coverage. This leads to a modular coil array system suitable for multiple application scenarios, eliminating the need for multiple expensive coils for each anatomical region. These combined features position them as a groundbreaking advancement in the realm of MRI technology.
What do you hope to study next?
In future studies, we aim to translate these coils into clinical practice to ensure the new technology is effectively integrated into clinical scenarios and improves patient outcomes.
We will collaborate with health care professionals, including physicians and technicians, to gather insights and define the clinical problems this technology aims to address.
Based on specific clinical requirements, such as the anatomical configurations of disease-related sites, the penetration depth of abnormalities, and the desired area of the region of interest, we will further customize the coil design, including their size, configurations, and assembly of units in metamaterials.
Through an iterative process, we aim to develop advanced MRI coils that can be seamlessly integrated into everyday health care settings and existing clinical workflows to improve patient care.
More information:
Ke Wu et al, Computational‐Design Enabled Wearable and Tunable Metamaterials via Freeform Auxetics for Magnetic Resonance Imaging, Advanced Science (2024). DOI: 10.1002/advs.202400261
Xia Zhu et al, Wearable Coaxially‐Shielded Metamaterial for Magnetic Resonance Imaging, Advanced Materials (2024). DOI: 10.1002/adma.202313692
Ke Wu et al, Wireless, Customizable Coaxially-shielded Coils for Magnetic Resonance Imaging, Science Advances (2024). DOI: 10.1126/sciadv.adn5195. www.science.org/doi/10.1126/sciadv.adn5195 . On arXiv: arxiv.org/abs/2312.12581
Citation:
Engineer explains the science behind metamaterials and MRI enhancement (2024, June 12)
retrieved 12 June 2024
from https://phys.org/news/2024-06-science-metamaterials-mri.html
This document is subject to copyright. Apart from any fair dealing for the purpose of private study or research, no
part may be reproduced without the written permission. The content is provided for information purposes only.
Discussion about this post