In 1906, pathologist Camillo Golgi and neuroscientist Santiago Ramón y Cajal won the Nobel Prize for their work on the structure of the nervous system. More than a century later, the puzzle of nervous system organization—the intricately tangled mess that results from each neuron’s connections to thousands of others—remains incomplete.
Neuroscientist Edward Callaway uses monosynaptic rabies virus tracing to map neuronal circuits in the cortex.
Chris Keeney
Yet, fully developing scientific understanding of these connections is crucial, said Edward Callaway, a systems neurobiologist at the Salk Institute. “If you don’t have some knowledge about how the different parts are interacting, there’s no way to generate a hypothesis about how they’re working together.”
Disentangling this complex network to create an orderly map of neuronal connections is a problem that researchers have been working on for decades. In the 1980s, researchers began searching for a marker that could function as a transneuronal tracer. They needed something that could hop across synapses, spreading from neuron to neuron efficiently enough to reach detectable levels even in cells connected across long distances. Luckily for the scientists (although perhaps unluckily for humanity in general), a handful of neuron-specializing viruses have evolved to do exactly that.
Thanks to the work of pioneering virologists, neuroscientist Gabriella Ugolini, now at the Paris-Saclay Institute of Neuroscience, knew that herpes simplex virus 1 (HSV-1) preferentially infected neurons, and that it could spread across synaptic connections. Unlike a dye or a toxin, a virus would not need to cross synapses in large amounts because it could replicate to detectable levels using the host’s cellular machinery. In the late 1980s, she demonstrated that when injected into a peripheral nerve, HSV-1 spread across synapses to neurons in the brain.1 The virus-infected neurons could then be easily identified in sections of brain tissue using immunohistochemistry, providing a clear picture of the chains of connection.
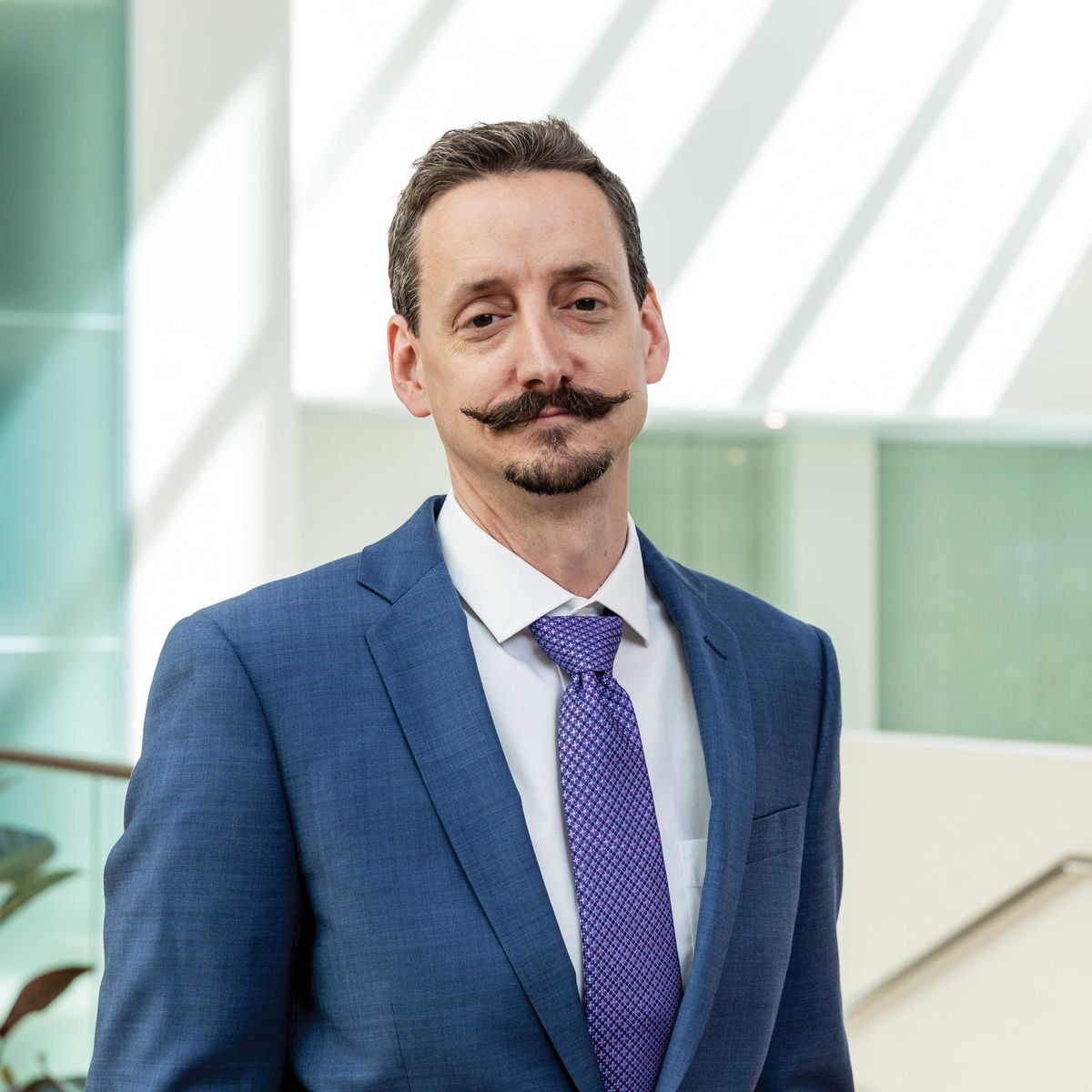
Neuroscientist Ian Wickersham develops next-generation rabies tracing techniques to enable long-term studies.
Caitlin Cunningham/McGovern Institute
By the early 2000s, several researchers used herpes and rabies, another neurotropic virus, to map connections within the brain. Peter Strick, currently a neuroscientist at the University of Pittsburgh, was mapping the motor circuit between the basal ganglia and the cortex, and Rockefeller University molecular geneticist Jeffrey Friedman had mapped inputs to the hypothalamus using the first cell type specific viral tracing system.2,3
These viral systems worked—almost too well, in fact. Once a virus began to replicate, it would spread from cell to cell to cell without stopping. “There was no way of unambiguously determining how many synapses had been crossed,” said Ian Wickersham, who now researches genetic neuroengineering at the Massachusetts Institute of Technology (MIT). “The virus spreads across different synapses at different rates, depending on how much hardware there is to support the spread across that particular synapse.”
So, Callaway and Wickersham (at the time a graduate student in Callaway’s lab) set out to develop a tracer virus that could only establish an initial infection in a defined subset of neurons. Then, after proliferating in these “starter” cells, the virus would only cross one synapse, thus labeling only the starter cell and the cells directly connected to it.
See also: https://www.the-scientist.com/a-cell-by-cell-map-of-the-entire-mouse-brain-71679
Going Viral
Since Friedman had already developed a type of herpesvirus, Ba2001, that could only infect specific cell types, Wickersham first attempted to modify this virus so that it could travel across only one synapse.3 However, the researchers repeatedly ran into problems with toxicity: The virus killed the neurons too quickly.
“We generally just saw tissue that looked crappy and dead,” recalled Callaway.
If you don’t have some knowledge about how the different parts are interacting, there’s no way to generate a hypothesis about how they’re working together.
—Edward Callaway, the Salk Institute
They went back to the drawing board and started over with rabies virus, a much slower killer, which naturally crosses synapses solely in the retrograde direction. Rabies virus, however, presented its own challenges. To transform the wild-type virus into a cell type-specific, monosynaptic tracer, they needed to modify its genome. This was no easy task to accomplish, as contemporary genome modification strategies largely targeted DNA, and rabies is a negative strand RNA virus. In searching through the scientific literature, Wickersham discovered that someone had already solved this problem. Karl-Klaus Conzelmann, a virologist at the University of Munich, had figured out how to delete the rabies’ spike glycoprotein gene, which enables the virus to spread from one neuron to another.4,5
This modified virus was the perfect starting point for their project, and fortunately Conzelmann was willing to share his creation with the team at the Salk Institute. By transfecting the starter neurons with a plasmid containing the missing glycoprotein gene, the researchers ensured the glycoprotein’s presence in these cells. The virus could then use this available glycoprotein to make infectious copies of itself, building the glycoprotein-coated envelope that it needed to enter synaptically-connected neurons.
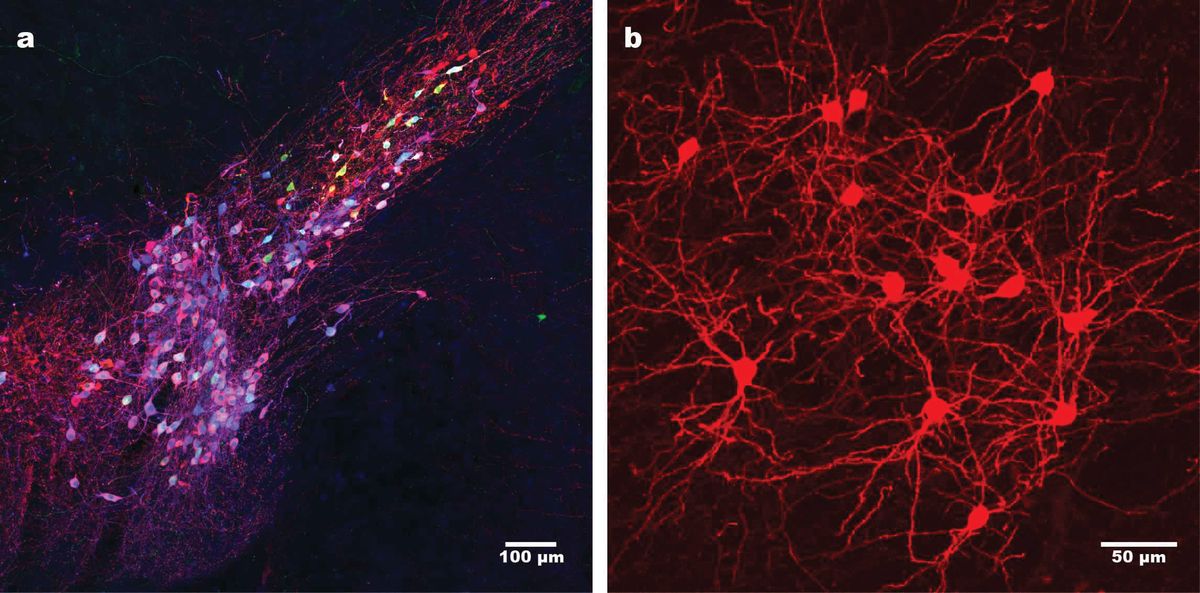
Scientists use second-generation monosynaptic tracing to label dopamine-expressing neurons (blue) in the mouse substantia nigra (left) and neurons in the striatum (right) that provide input to these cells. Starter cells are shown in green; cells containing the virus are shown in red.
Ian Wickersham and Lei Jin
Upon entering the presynaptic neurons, the virus is uncoated, losing its glycoprotein envelope to release the genome for transcription. The virus can replicate in these secondary neurons, which is a helpful trait for scientists hoping to track a fluorescent signal. However, since these cells have not been engineered to produce glycoprotein, and the modified virus does not contain the glycoprotein gene, it cannot build infectious copies of itself, and thus, it cannot spread any farther.
This was only half the battle, however. They still needed to figure out how to ensure that the initial infection occurred only in a defined population of starter cells. Serendipitously, Callaway happened to mention their conundrum to a fellow Salk Institute scientist, microbiologist John Young.
As a postdoctoral researcher in the early 1990s, Young had studied a virus called subgroup A avian sarcoma and leukosis virus (ASLV-A), with a particular focus on how viral entry was mediated by interactions between the viral envelope protein (EnvA) and an avian cell surface protein called tumor virus receptor A (TVA). This highly specific lock and key system, which did not naturally exist in mammalian tissues, was exactly what the researchers needed to target a defined population of starter cells.
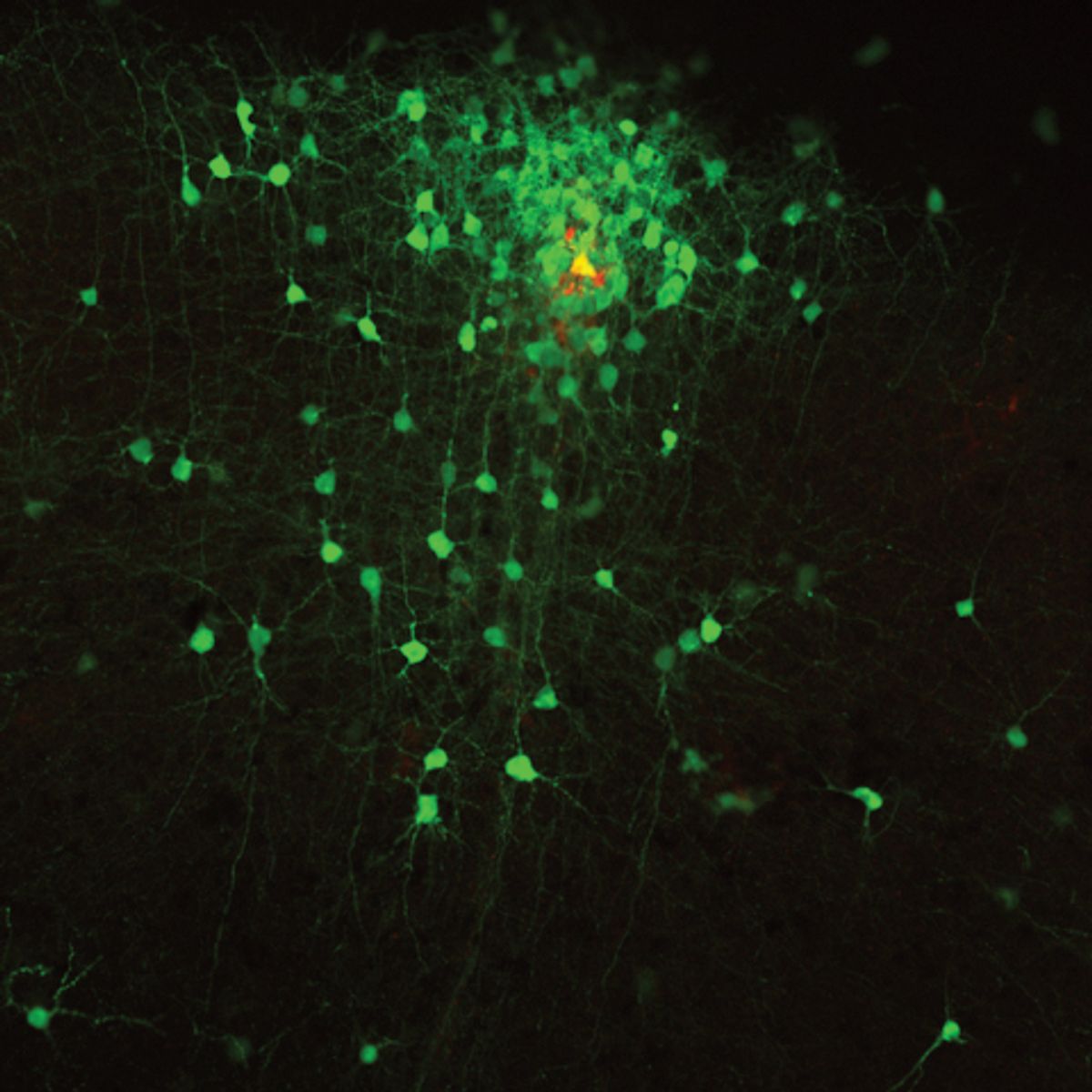
A single “starter” neuron (shown in yellow) connects to a multitude of other neurons (shown in green).
Euiseok Kim
“I really can’t get over how lucky it was that John had literally, in his previous lab, found the perfect solution to this problem,” said Wickersham.
Finally, after years of experiments, the team was ready to put all the puzzle pieces together. Working with slices of brain tissue, they transfected starter neurons with plasmids encoding the rabies glycoprotein and the TVA receptor. Then they introduced the tracer, a green fluorescent rabies virus that lacked its native glycoprotein and was neatly packaged in a viral envelope studded with EnvA from the avian virus.
As the researchers had hoped, the tracer’s EnvA key fit perfectly into the TVA receptors on the starter cells, but was unable to begin an infection in any cells without this bird protein. By taking advantage of the glycoprotein in the starter cells, the virus could make infectious copies of itself, which spread to every presynaptic neuron connected to the starter cell. Once in these connected cells, the virus found itself without glycoprotein and could spread no farther, ultimately labeling each starter cell and its direct connections a beautifully bright fluorescent green.6
Guns, Germs, and…Brains
In their first proof-of-concept publication, researchers used a technique called biolistic transfection for the initial transfection step. “[We used] a so-called gene gun, which is basically just like a compressed air gun, which shoots these little, tiny pellets of gold coated with DNA physically into cells,” said Wickersham.
This was never meant to be the final version of the technique, however. The gene gun couldn’t be used in vivo and limiting the work to brain slices that are generally less than a millimeter thick would make it extremely difficult to identify distantly connected neurons.
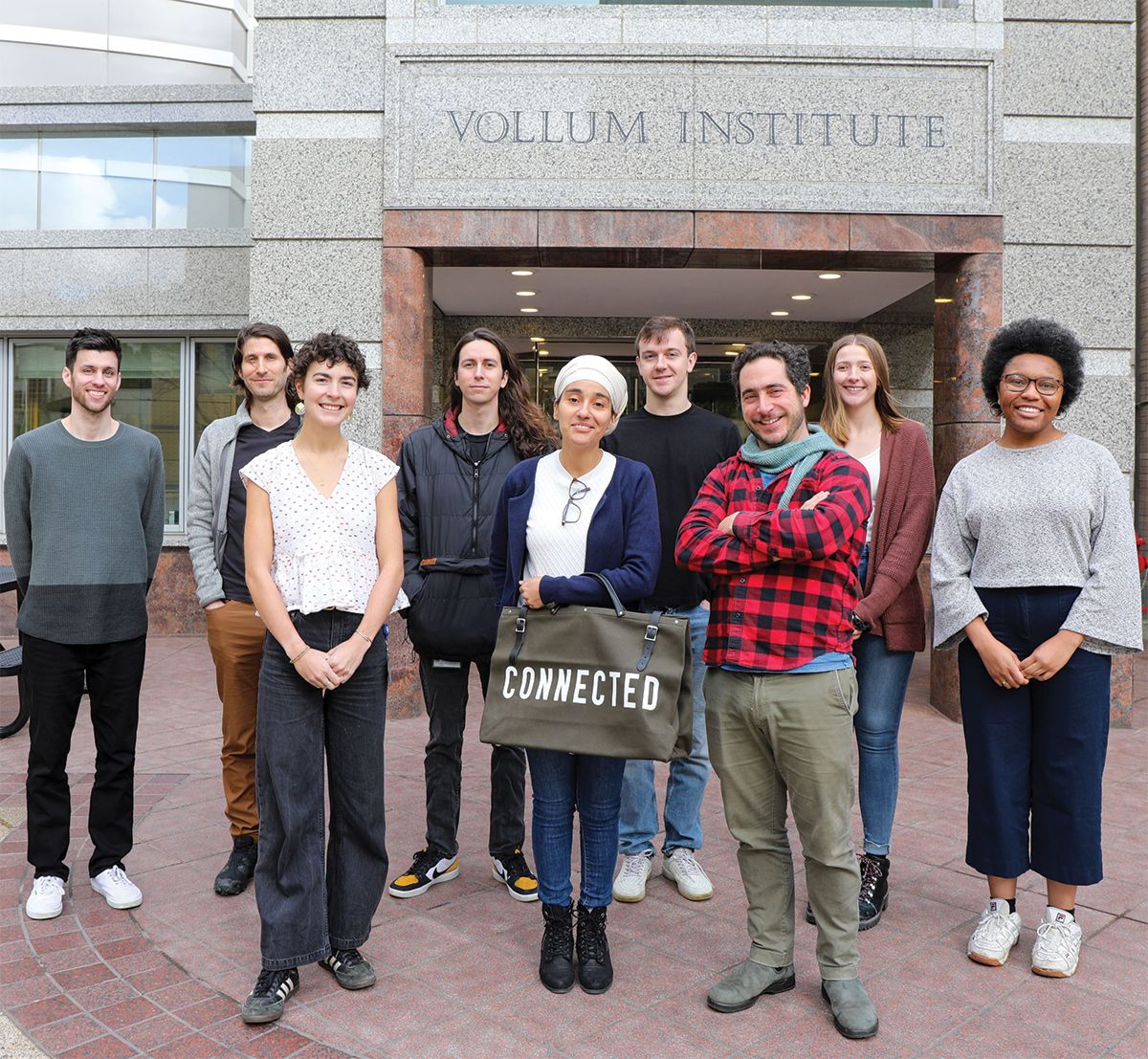
Researchers in the Saunders Lab use neurotropic viruses to map neural circuits.
Christine Torres Hicks (OHSU)
To overcome this problem, the researchers turned to the rapidly expanding world of Cre transgenic mouse lines. Instead of using the gene gun to deliver the TVA receptor and rabies glycoprotein genes to the starter neurons, they developed a Cre-dependent adeno-associated virus (AAV) helper virus, which, when injected into the brain, resulted in the expression of these two genes only in neurons that also expressed Cre recombinase. Afterwards, the modified rabies virus was injected, resulting in the same monosynaptic anterograde tracing, this time in a living animal.7
While this version of the rabies-based transsynaptic tracing technique is still widely used today, Callaway, Wickersham, and others have greatly expanded this tool’s original capabilities. Callaway’s research group demonstrated that this technique could be used not only to label connected neurons, but also to deliver tools to report, suppress, or enhance neuronal activity and manipulate gene expression.8 Wickersham’s group at MIT developed a second-generation system with reduced toxicity, enabling long-term labeling of connected neurons without killing the cells or even substantially affecting their function.9 Now, their research groups as well as others, hope to take the technique even farther.
See also: https://www.the-scientist.com/a-novel-panic-pathway-in-the-brain-71837
Scaling up to Study Connectopathies
While the original Cre-dependent technique remains useful for scientists studying broad cell types like glutamatergic or dopaminergic neurons, it is insufficient to fully capture the incredible complexity of the brain. Even the mouse brain, which is relatively simple compared to a human brain, is thought to contain more than 5,000 different cell types.
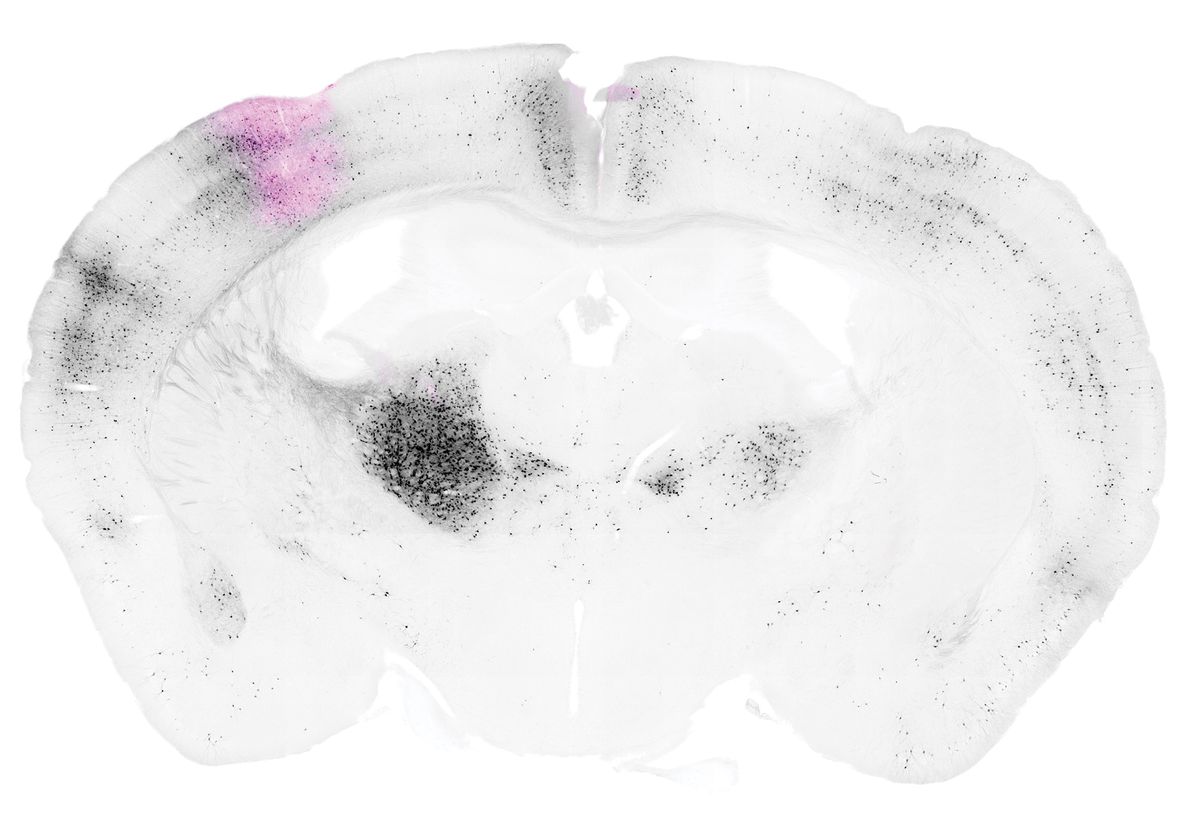
Modified rabies virus reveals connections between “starter” cells in the mouse primary sensory cortex (pink) and neurons in many other brain regions (black).
Austin Schubert
To capture this remarkable diversity and create more comprehensive maps of neural connectivity, researchers are combining rabies-based transsynaptic tracing with single-cell RNA sequencing.
As a postdoctoral researcher in neurobiologist Steven McCarroll’s Harvard Medical School research group, Arpiar Saunders became well-versed in single-cell RNA sequencing, creating RNA expression maps containing hundreds of thousands of brain cells.10 Saunders, now a neurophysiologist at Oregon Health and Science University, said, “The next question was: after we know the building blocks of the brain, how do we [figure out] how those building blocks assemble into neural circuits?”
To answer this question, Saunders and McCarroll developed the synaptic barcode analysis by retrograde rabies readout method, also known as SBARRO, which analyzes the viral and host cell RNA.11 Using SBARRO, said Saunders, “You can reconstruct the network that the individual cell was part of and also the molecular type of that cell.” In order to capture many individual networks in one experiment, they generated a library of rabies viruses, each containing a 20-base-pair barcode. This allowed researchers to identify connected cells by matching their viral barcodes.
In the first demonstration of this technique, researchers used brain cell networks grown in culture, but Saunders is currently working on applying this viral barcode technique to intact brain tissues in mice and eventually in nonhuman primates. Using a somewhat different approach, Wickersham is also developing a synaptic mapping technique for intact brain tissue using barcoded rabies virus and in situ sequencing in collaboration with neuroscientist Xiaoyin Chen at the Allen Institute for Brain Research.12
There’s been a number of techniques in biology that have had major impact by essentially taking a very complex problem and breaking it into fundamental units that are easy to measure.
—Arpiar Saunders, Oregon Health and Science University
“There’s been a number of techniques in biology that have had major impact by essentially taking a very complex problem and breaking it into fundamental units that are easy to measure,” said Saunders. In this case, each unit consists of one cell and all its presynaptic connections. While there are still methodological challenges to be solved, Saunders hopes that these techniques will allow scientists to identify these units, which can eventually be computationally assembled into a comprehensive brain wiring diagram.
“Within the next couple years—[for] our group and probably other groups as well—the aim is to image thousands of monosynaptic networks in an intact mouse brain to give us a very quantitative picture of the synaptic organization of neural circuits. It sounds kind of crazy, and it’s aspirational,” said Saunders. “But I’m optimistic that we can achieve it.”
Creating the wiring diagrams is only the first step, however. “We’re really interested in how neurodevelopmental disorders might be disorders of neural wiring, or connectopathies,” said Saunders.13 “There is striking genetic evidence that mutations associated with autism and schizophrenia are enriched in genes that encode synaptic proteins. We also know from very hypothesis-driven, focused work on particular synapses that there are changes [in these disorders]. But we don’t have any way yet to build big picture, aerial descriptions of the type of connectivity changes that might be occurring, so that is our goal.”
References
- Ugolini G et al. Transneuronal transfer of herpes virus from peripheral nerves to cortex and brainstem. Science. 1989;243(4887):89-91.
- Kelly RM, Strick PL. Macro-architecture of basal ganglia loops with the cerebral cortex: use of rabies virus to reveal multisynaptic circuits. Prog Brain Res. 2004;143:449-459.
- DeFalco J et al. Virus-Assisted Mapping of Neural Inputs to a Feeding Center in the Hypothalamus. Science. 2001;291(5513):2608-2613.
- Mebatsion T et al. Budding of rabies virus particles in the absence of the spike glycoprotein. Cell. 1996;84(6):941-951.
- Etessami R et al. Spread and pathogenic characteristics of a G-deficient rabies virus recombinant: an in vitro and in vivo study. J Gen Virol. 2000;81(Pt 9):2147-2153.
- Wickersham IR et al. Monosynaptic restriction of transsynaptic tracing from single, genetically targeted neurons. Neuron. 2007;53(5):639-647.
- Wall NR et al. Monosynaptic circuit tracing in vivo through Cre-dependent targeting and complementation of modified rabies virus. Proc Natl Acad Sci U S A. 2010;107(50):21848-21853.
- Osakada F et al. New rabies virus variants for monitoring and manipulating activity and gene expression in defined neural circuits. Neuron. 2011;71(4):617-631.
- Jin L et al. Long-term labeling and imaging of synaptically connected neuronal networks in vivo using double-deletion-mutant rabies viruses. Nat Neurosci. 2024;27(2):373-383.
- Saunders A et al. Molecular Diversity and Specializations among the Cells of the Adult Mouse Brain. Cell. 2018;174(4):1015-1030.e16.
- Saunders A et al. Ascertaining cells’ synaptic connections and RNA expression simultaneously with barcoded rabies virus libraries. Nat Commun. 2022;13(1):6993.
- Zhang A et al. Rabies virus-based barcoded neuroanatomy resolved by single-cell RNA and in situ sequencing. Elife. 2024;12:RP87866.
- van den Heuvel MP, Sporns O. A cross-disorder connectome landscape of brain dysconnectivity. Nat Rev Neurosci. 2019;20(7):435-446.
Discussion about this post