Article reviewed by Huiwang Ai, PhD from the University of Virginia.
Stay up to date on the latest science with Brush Up Summaries.
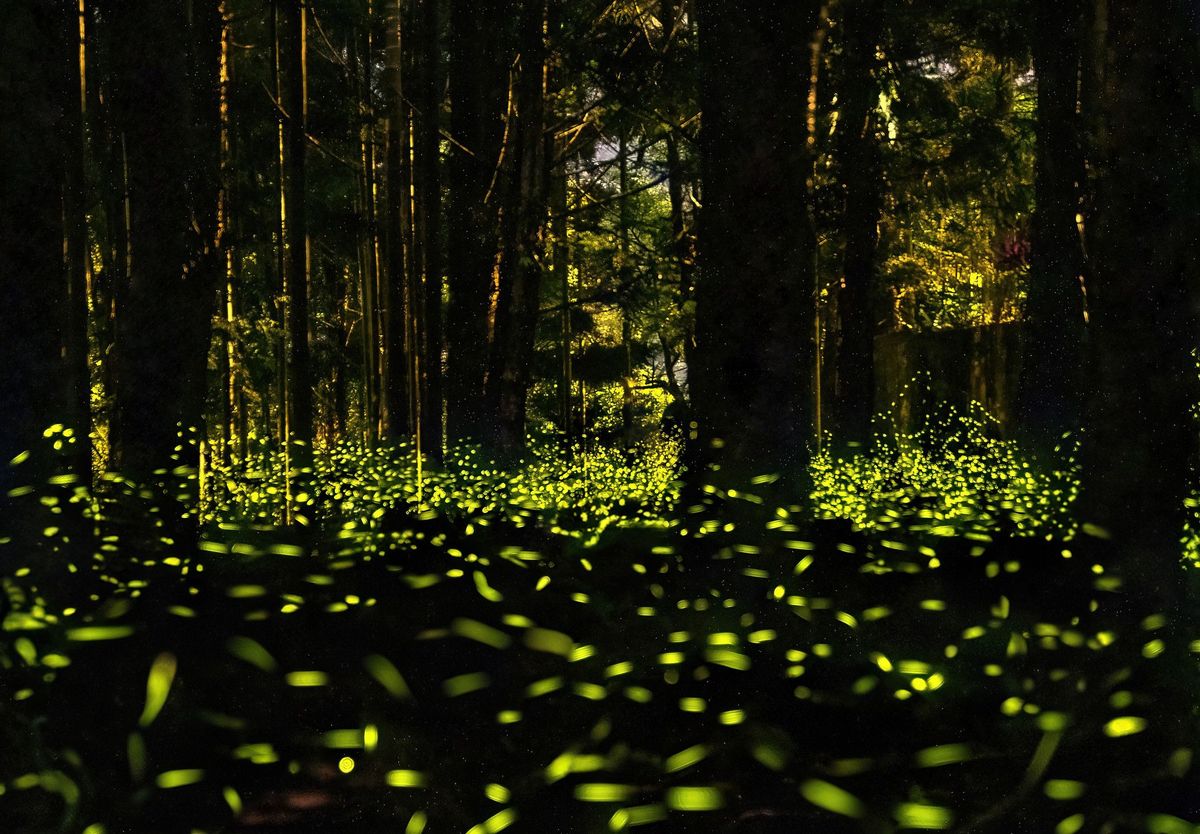
Glowing fireflies illuminate a forest with the help of luciferase enzymes.
What Is Luciferase?
Luciferase is a group of enzymes that oxidize a substrate known as luciferin to produce light.1 For bioluminescence, the firefly luciferase enzyme catalyzes the oxidation of D-luciferin in the presence of oxygen (O2), adenosine triphosphate (ATP), and magnesium ions (Mg2+). This catalytic reaction converts the excited oxyluciferin molecule to its ground state, emitting visible light. Bioluminescence observed in various organisms may utilize different chemical processes. However, they all share a common feature: the production of light through enzyme-catalyzed oxidation reactions.
History of Luciferase Enzymes
Although bioluminescence has been observed for many centuries, its application in biomedicine is relatively recent. Scientists traced back the first reference of bioluminescence to the Greek philosopher Aristotle in 384-322 BCE.2 Almost three centuries later, Gaius Plinius Secundus, a Roman author, conducted an in-depth study on bioluminescence. He discovered many animals that had illuminating capacity such as purple jellyfish, mollusks, fireflies, and glowworms.
French pharmacologist Raphael Dubois observed that a specific constituent of the click beetle was essential for bioluminescent reactions. He extracted two components from beetle abdomens, naming the substrate luciferine and the enzyme responsible for the reaction luciferase.2
Over many years, scientists across the world conducted multiple experiments and established that luciferase enzymes and substrates are species-specific. For example, the Gaussia and Renilla luciferases use coelenterazine as their substrate to emit blue to cyan light, while Cypridina uses its own genus-specific luciferin to generate blue light.3,4 In 1985, scientists made a milestone scientific breakthrough by cloning the firefly luciferase (FLuc) in Escherichia coli, enabling an unlimited source for this enzyme.
Luciferase Sources and Types
Different types of luciferases are present in aquatic and terrestrial species including insects, bacteria, and fungi.2 Scientists estimated that around 75% of sea animals are bioluminescent.5
In nature, a luciferin–luciferase system is present in the firefly (Photinus pyralis), bacteria (Vibrio harveyi), sea pansy (Renilla reniformis), jellyfish (Aequorea victori), ostracod (Cypridina noctiluca), dinoflagellates (Gonycaulax), and copepod (Gaussia princeps).6
Not all bioluminescent marine organism systems can be used for animal imaging because the majority of them generate blue light that does not penetrate tissue appropriately for detection. Furthermore, certain small molecule substrates of marine organisms are unstable and easily oxidized without luciferase.
Table 1: Different types of luciferase and their applications7
Luciferase |
Organism |
Substrate |
Maximum emission wavelength (nm) |
Firefly luciferase (FLuc) |
Photinus pyralis (Firefly) |
D-Luciferin |
560 |
Renilla luciferase (RLuc) |
Renilla reniformis (Sea pansy) |
Coelenterazine |
480 |
NanoLuc luciferase (NLuc) |
Engineered |
Furimazine |
460 |
Gaussia luciferase (GLuc) |
Gaussia princeps (Palma de sierra) |
Coelenterazine |
470 |
Bacterial luciferases (Lux) |
Vibrio harveyi |
Reduced riboflavin phosphate |
490 |
Fungal luciferase (Luz) |
Neonothopanus nambi |
Luciferin |
520 |
Akaluciferase (AkaLuc) |
Engineered |
Akalumine |
677 |
Among the different types of luciferases, scientists use FLuc widely for biological experiments because of its high quantum yield, elevated signal to noise ratio, and magnificent color. Furthermore, these luciferases trigger yellow-green to red light emission, depending on reaction conditions, which has a higher cellular or tissue penetrance. Luciferin is biologically stable as it is not oxidized in the absence of the enzyme.
Biochemical engineers created several generations of FLuc that exhibit improved brightness and other properties. For example, Luc2 is a second-generation firefly luciferase with an optimized codon that facilitates its expression in mammalian systems.8 The new firefly bioluminescence system Akaluciferase (AkaLuc)-AkaLumine produces greater signal strength than the standard Fluc-luciferin reaction.9 AkaLuc-AkaLumine (named after the Japanese word for the color red, aka) is a non-invasive and highly sensitive bioluminescence system that emits red light in the near-infrared (NIR) range for better tissue penetration. Besides FLuc, researchers also use NanoLuc luciferase (NLuc) and Renilla luciferase (RLuc) for biological experiments.10,11
Each luciferase type has different advantages and disadvantages depending on the application.12 For instance, both FLuc and RLuc have short protein half-lives making them favorable transcriptional reporters. Even though FLuc is brighter than RLuc, the former is more vulnerable to enzyme inhibition. Because FLuc and RLuc are not secreted, transfected cells must be lysed, followed by intracellular substrate introduction and luminescence measurement. Furthermore, both FLuc and RLuc are large, making them less favorable protein tags.
The secreted Gaussia luciferase (GLuc) is more stable.13 However, a relatively short luminescence half-life makes it unsuitable for many experimental settings. NLuc is an engineered luciferase derived from deep sea luminescent shrimp. This reporter gene has significantly attracted scientists for its greater stability, smaller size, and >150-fold increase in luminescence. Despite these benefits, they are not ideal for in vivo applications because its blue emission and their substrate (furimazine) has low solubility and bioavailability.
The major advantage of a bacterial bioluminescence system is that, since researchers have figured out the genetic pathways to synthesize all of the substrates required for the emission of light, no exogenous luciferin addition is needed.14 However, its dependence on reduced riboflavin phosphate (FMNH2), which is available at a limited quantity in mammalian cells, and low intensity blue light emission makes it less favorable for eukaryotic systems. In addition, fungi possess a bioluminescent system with the synthetic genes elucidated, but the intensity of this system is still weak.15
Luciferase Reporter Assay
Luciferase reporter assays help determine whether a protein activates or suppresses transcription of the target gene. It also analyzes translation regulation by cis-elements of an mRNA present in the 5′-untranslated region (UTR) and 3′-UTR.
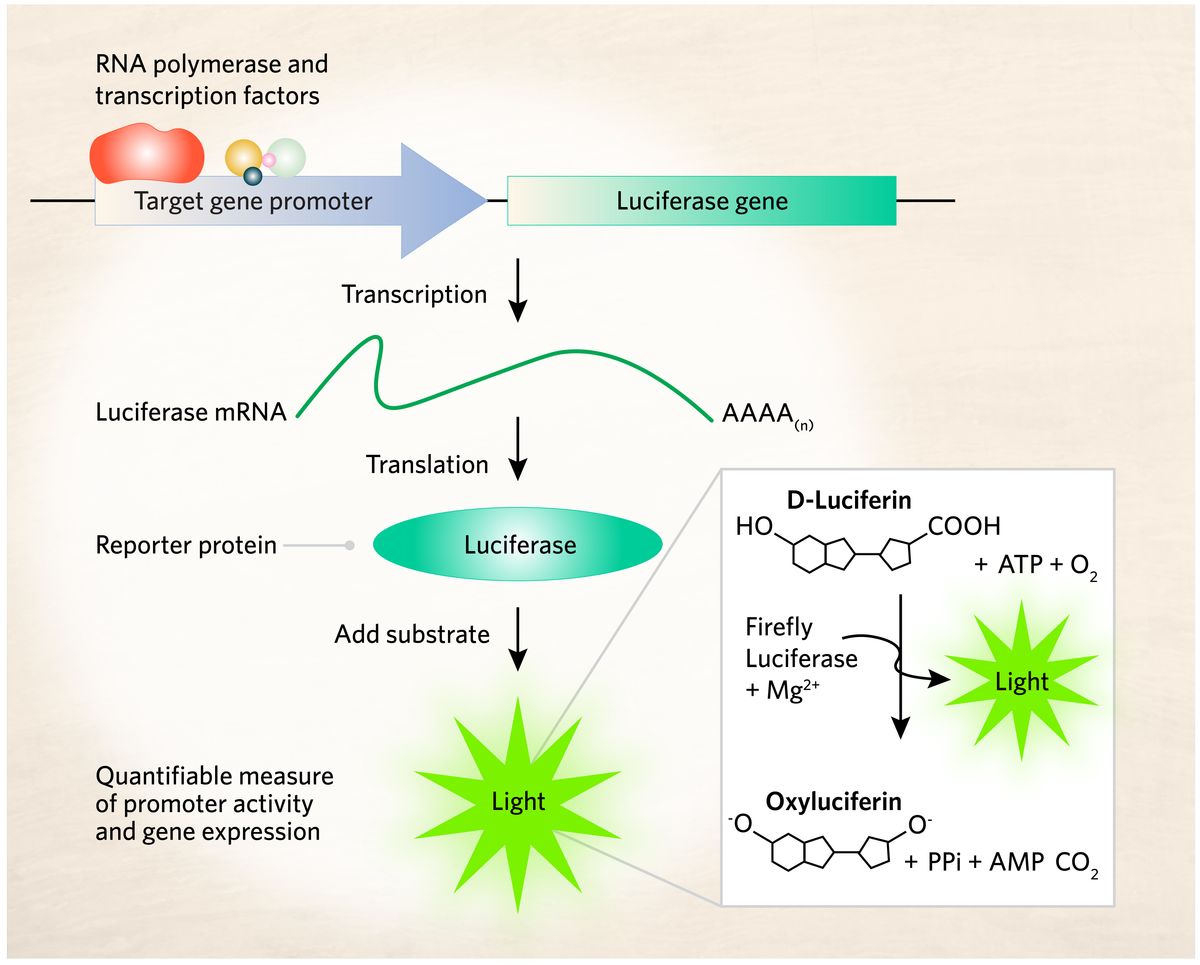
Luciferin reporter assays employ a luciferase-luciferin light emitting reaction as a readout for target gene promoter activity and gene expression.
The Scientist
Scientists use recombinant DNA technology to develop promoter-reporter constructs.16 They fuse the regulatory region of the target gene with a luciferase reporter gene. A second DNA construct encodes proteins hypothesized to influence transcription. Researchers transfect a cell culture system such as HEK 293T cells with both constructs.17
Typically, scientists examine luciferase expression two to three days after cell transfection. They lyse the transfected cells and place the contents in a reaction tube. Researchers add a suitable substrate to the reaction tube, such as luciferin, which enables luciferase to catalyze a chemical reaction that produces light. A luminometer can detect and quantify the amount of light produced in each reaction tube.18 The light intensity indicates the amount of target gene expression. If a protein upregulates target gene transcription, the cell expresses more luciferase molecules, which generates bright light. However, if the protein downregulates transcription, negligible luciferase expression occurs.
Single luciferase reporter assay
Promoter analysis in bacteria or eukaryotic cells typically employs a single luciferase reporter assay.19 This assay requires an excess of luciferin and ATP. A single luciferase reporter assay follows the classical methodology where scientists transfect cells with a promoter-reporter construct, followed by lysis, substrate introduction, and detection. Besides gene expression analysis, this assay is suitable for high throughput screening of protein-protein interactions as well.
Scientists designed the split luciferase complementation (SLC) method to study protein-protein interactions.20 This assay determines the effect of the physical contact between signaling proteins. The SLC method detects the factor that inhibits protein-protein interactions. It involves the genetic fusion of two inactive luciferase fragments with interacting proteins. The strongest luminescence corresponds to intense interactions between the protein pair and vice versa.
Dual luciferase assay
Dual luciferase assays exploit the varying biochemical luminescence requirements of different species’ luciferase proteins.21 They allow the sequential quantitative measurement of FLuc and RLuc activities in a single protein extract.
Scientists use this method to simultaneously monitor different cellular events and their regulation based on two distinct signal emissions (e.g., green and red light). The improvement in DLR applicability relies on the availability of a luminometer equipped with two optical filters. For example, researchers developed a DLR assay using two reporter genes (FLuc and RLuc) that quantifies sequential gene expression in yeast (Saccharomyces cerevisiae) using an advanced luminometer with wavelength-specific filters offering exceptional sensitivity for detecting distinct emissions. 22
Multicolored luciferase reporter assay
Multicolor luciferase reporter assays are powerful molecular biological tools that enable simultaneous monitoring of multiple genes with a single substrate.23 Scientists designed reporter genes for multicolor luciferase assays using three separate luciferase genes that emit green, orange, and red light upon addition of a single substrate. This method’s major advantage is the ability to monitor sequential and complex expression changes in multiple genes within a single cell or tissue. Researchers apply this assay for high-throughput gene expression analysis.
Current Research Outlook
A luciferase-substrate reaction emits light ranging across many wavelengths. The sensitivity of in vivo bioluminescence imaging depends on light emission at the NIR range (700-900nm) and tissue permeability of the number of photons emitted. Scientists developed a NIR luciferin analog to detect photons from deep target tissues with high sensitivity.24 Currently, many researchers are focusing on tuning the color of light emitted by bioluminating reporter genes towards the NIR or IR region, which has better tissue penetrance.
Scientists are also working on multicomponent imaging to study several features or cell types simultaneously. The main concept behind this technique is the selective introduction of different luciferase molecules into separate cells within the same system. Upon specific substrate addition, only target cells light up. Scientists developed a multicolor bioluminescence system using NIR-emitting luciferases that enabled detection of two different cell populations within one animal model.25 A key shortcoming of this technique is the lack of a standardized method to visualize combinations of luciferase reporters.
- Vysotski ES. Bioluminescent and fluorescent proteins: Molecular mechanisms and modern applications. Int J Mol Sci. 2022;24(1):281.
- England CG, et al. NanoLuc: A small luciferase is brightening up the field of bioluminescence. Bioconjug Chem. 2016;27(5):1175-1187.
- Jiang T, et al. Coelenterazine-type bioluminescence-induced optical probes for sensing and controlling biological processes. Int J Mol Sci. 2023;24(6):5074.
- Kanie S, et al. Luminescence of Cypridina luciferin in the presence of human plasma alpha 1-acid glycoprotein. Int J Mol Sci. 2020;21(20):7516.
- Martini S, Haddock SH. Quantification of bioluminescence from the surface to the deep sea demonstrates its predominance as an ecological trait. Sci Rep. 2017;7:45750. Published 2017 Apr 4.
- Greer LF, Szalay AA. Imaging of light emission from the expression of luciferases in living cells and organisms: A review. Luminescence. 2002;17(1):43-74.
- Fleiss A, Sarkisyan KS. A brief review of bioluminescent systems. Curr Genet. 2019;65:877-882.
- Levin RA, et al. An optimized triple modality reporter for quantitative in vivo tumor imaging and therapy evaluation. PLoS One. 2014; 9(5):e97415.
- Ito A, et al.Akaluc/AkaLumine bioluminescence system enables highly sensitive, non-invasive and temporal monitoring of gene expression in Drosophila. Commun Biol. 2023; 6, 1270.
- Boute N, et al. NanoLuc luciferase – A multifunctional tool for high throughput antibody screening. Front Pharmacol. 2016;7:27.
- Woo J, et al. Structure-function studies on the active site of the coelenterazine-dependent luciferase from Renilla. Protein Sci. 2008;17(4):725-735.
- Thorne N, et al. Illuminating insights into firefly luciferase and other bioluminescent reporters used in chemical biology. Chem Biol. 2010;17(6):646-657.
- Tannous BA. Gaussia luciferase reporter assay for monitoring biological processes in culture and in vivo. Nat Protoc. 2009;4(4):582-591.
- Brodl E, et al. Molecular mechanisms of bacterial bioluminescence. Comput Struct Biotechnol J. 2018;16:551-564.
- Kotlobay AA, et al. Genetically encodable bioluminescent system from fungi. PNAS. 2018;115(50):12728-12732.
- Gerlach RG, et al. Rapid engineering of bacterial reporter gene fusions by using Red recombination. Appl Environ Microbiol. 2007;73(13):4234-4242.
- Carter M, Shieh J. Biochemical assays and intracellular signaling. In: Guide to Research Techniques in Neuroscience (Second Edition). 2015;311-343.
- Klein MA, et al. Luciferase calibrants enable absolute quantitation of bioluminescence power. ACS Meas Sci Au. 2023;3(6):496-503.
- Barriscale KA, et al. A single secreted luciferase-based gene reporter assay. Anal Biochem. 2014;453:44-49.
- Lang Y, et al. Analysis of protein-protein interactions by split luciferase complementation assay. Curr Protoc Toxicol. 2019;82(1):e90.
- Branchini BR, et al. A firefly luciferase dual color bioluminescence reporter assay using two substrates to simultaneously monitor two gene expression events. Sci Rep. 2018;8(1):1-7.
- McNabb DS, et al. Dual luciferase assay system for rapid assessment of gene expression in Saccharomyces cerevisiae. Eukaryot Cell. 2005;4(9):1539-1549.
- Nakajima Y. Multicolor luciferase assay system: one-step monitoring of multiple gene expressions with a single substrate. BioTechniques. 2018;38(6).
- Kitada N, et al. Development of near-infrared firefly luciferin analogue reacted with wild-type and mutant luciferases. Chirality. 2020;32(7):922-931.
- Zambito G, Mezzanotte L. Near-infrared bioluminescence imaging of two cell populations in living mice. STAR Protoc. 2021; 2(3), 100662.