In 1986, Harold Dvorak, a pathologist at Harvard Medical School, popularized the idea of cancer as a wound that does not heal.1 In the following decades, many research groups drew intriguing parallels between processes involved in wound repair and cancer, including changes in the extracellular matrix, cell proliferation, and immune responses.2
However, much remains to be discovered. Yejing Ge, a cell biologist at the MD Anderson Cancer Center, discussed her research on skin-resident stem cells and the surprisingly similar process that drives these cells towards controlled tissue regeneration or unrestrained malignancy.
How did you become interested in the biology of skin stem cells?
My journey started when I was a postdoctoral fellow in Elaine Fuchs’ lab at Rockefeller University. I became interested in how adult stem cells help an organism sustain homeostasis. Most of the time, these stem cells aren’t doing much; they’re maintaining a low metabolism, and it’s almost like they are dormant. But under stress, like when the tissue is damaged, this reserve of stem cells springs into action. These multipotent adult stem cells self-renew and differentiate into all the cell types in a specific tissue, such as the skin or the gut or the blood.
Elaine is one of the pioneers who helped establish skin as a model for studying the functions of adult stem cells. During my fellowship with her, I started to study the mechanisms that influence these stem cell fate decisions in the skin.
What roles do skin stem cells play in wound healing and carcinogenesis?
When the skin is injured, quickly restoring barrier function is vital for the organism’s survival. The body calls on all the stem cells that are in the vicinity of the wound. It doesn’t matter if they’re hair follicle, epidermal, or glandular stem cells. During homeostasis, hair follicle stem cells only make cells in the hair follicle lineage, but after an injury, they expand their fate, gaining the ability to differentiate into epidermal cells as well. During my time in Elaine’s lab, we showed that this stem cell lineage infidelity is important for quickly restoring the skin barrier, but the twist is that cancer hijacks this exact process.3 In our mouse models, there were many similarities in the transcriptomes of the skin stem cells present in wounds and in squamous cell carcinomas.
In both cases, the stem cells coexpressed hair follicle lineage markers, like SRY-Box Transcription Factor 9 (SOX9), and epidermal lineage markers like Kruppel-like transcription factor 5 (KLF5). This lineage infidelity is required for malignancy. When we inactivated Sox9 or Klf5 in cancer initiating cells and transplanted them into immunocompromised mice, we blocked cancer development. In wound repair, this lineage infidelity gets resolved when the wound has healed, but in cancer, the cells seem to get stuck in this state.
Cancer is very smart, in a way; it finds the easiest path forward. Instead of inventing new pathways, it hijacks what the body already has. In this case, it’s accessing the body’s cellular program for wound repair.
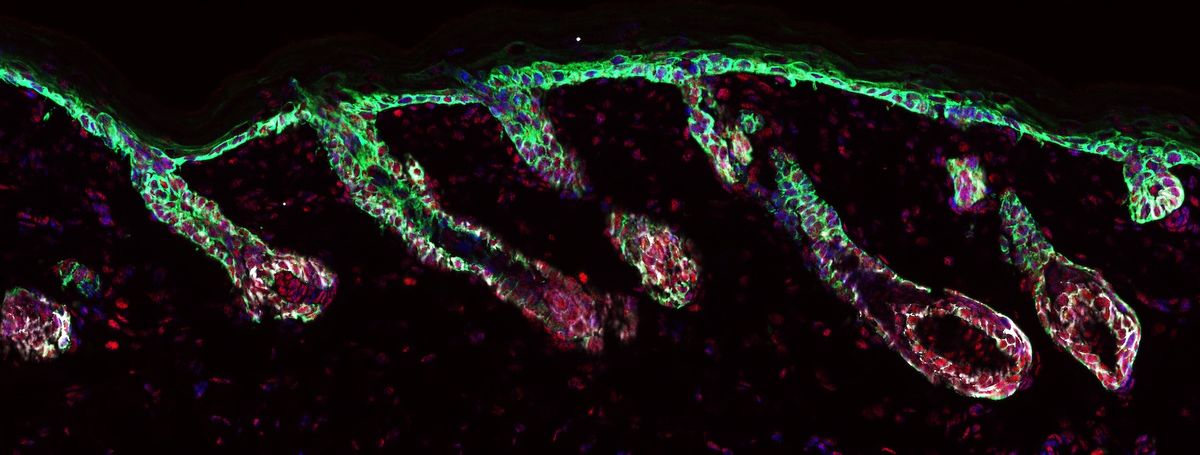
Hair follicle stem cells can expand their fates to produce epidermal cells to help wounds heal.
Ericka Humphrey
Could these findings help identify new therapeutic targets?
In our study, stress-activated transcription factors like E26 Transformation-Specific 2 (ETS2) are upregulated in wound repair and cancer. If we force the activation of this factor, we can mimic the lineage infidelity observed during these two processes. In wound repair, these transcription factors subside after the wound has healed, so the lineage infidelity is transient. In cancer, that doesn’t happen; oncogenic mutations or epigenetic alterations continue to drive expression of these transcription factors, locking the cell in this state of lineage infidelity.
Transcription factors are difficult to target and have historically been considered “undruggable” for many reasons, so these are not ideal.4 But as I’ve been starting my own lab, I’ve been thinking about ways to intercept this signaling and further explore this system to find vulnerabilities that we can therapeutically target. We know that the cell division-promoting pathways that get activated during wound repair are important for cancer development, but we don’t fully understand the epigenetic drivers of that signalling.
Coming back to stem cells, Shinya Yamanaka famously demonstrated that overexpressing certain transcription factors reprograms somatic cells into induced pluripotent stem cells.5 A key process in this transformation is the reactivation of the heterochromatin. There’s strong evidence that if we could somehow open the heterochromatin, then it would be possible to change cell fate. Thus, targeting epigenetic factors that change the accessibility of the chromatin could help regulate stem cell lineage plasticity and restore proper stem cell function.
What other factors can affect skin stem cell fates?
We started off by studying the changes that happen within these epithelial stem cells, but obviously these cells don’t live alone. They’re talking to other cells in their immediate niches, including fibroblasts and immune cells. When we put young stem cells in an aged environment, they lost their regeneration capacity; conversely, when we put aged stem cells in a young niche, it rescued their regeneration capacity.6 This isn’t to say that young and aged stem cells are exactly the same—they still have different epigenetic and transcriptional signatures—but at least in the short term, the microenvironment is dictating their behavior.
The aging microenvironment may also play an important role in cancer development. Most of us are walking around with oncogenic mutations, but for the most part, we don’t develop cancer. Researchers at the Sanger Institute sequenced normal skin and saw tons of somatic mutations in normal human skin at a frequency comparable to what you would see in skin cancer.7 So what is the mechanism that prevents these oncogenic events from giving rise to cancer? There’s some evidence that the microenvironment may be involved.
During aging, immune surveillance comes down and chronic inflammation develops; these are well-known factors that contribute to cancer. Certainly, immune cells in the skin, like dendritic cells and macrophages, are probably important for daily surveillance, but we are thinking about this phenomenon at multiple levels.
In an ongoing project, we’re comparing normal skin, squamous cell cancer, and actinic keratosis. The latter is a benign lesion, although about 10 percent of actinic keratosis cases will eventually progress to cancer. I want to know why some stem cells progress from benign to malignant lesions. We’re performing single-cell RNA sequencing on skin biopsies from patients, paying close attention to the epithelial cells but also looking at all the cells in the microenvironment. For each patient, we are comparing their cells from the lesion to those from healthy samples of their skin. So far it has been a very interesting adventure. I’m curious to see how our observations in mice will compare to what we see in humans; we think that a lot of the mechanisms are conserved, but specific molecules or interactions may differ.
References
- Dvorak HF. Tumors: Wounds that do not heal. Similarities between tumor stroma generation and wound healing. N Engl J Med. 1986;315(26):1650-1659.
- Ribatti D, Tamma R. A revisited concept. Tumors: Wounds that do not heal. Crit Rev Oncol Hematol. 2018;128:65-69.
- Ge Y et al. Stem cell lineage infidelity drives wound repair and cancer. Cell. 2017;169(4):636-650.e14.
- Tao Z, Wu X. Targeting transcription factors in Cancer: From “undruggable” to “druggable.” Methods Mol Biol. 2023;2594:107-131.
- Takahashi K, Yamanaka S. Induction of pluripotent stem cells from mouse embryonic and adult fibroblast cultures by defined factors. Cell. 2006;126(4):663-676.
- Ge Y et al. The aging skin microenvironment dictates stem cell behavior. Proc Natl Acad Sci U S A. 2020;117(10):5339-5350.
- Martincorena I et al. Tumor evolution. High burden and pervasive positive selection of somatic mutations in normal human skin. Science. 2015;348(6237):880-886.